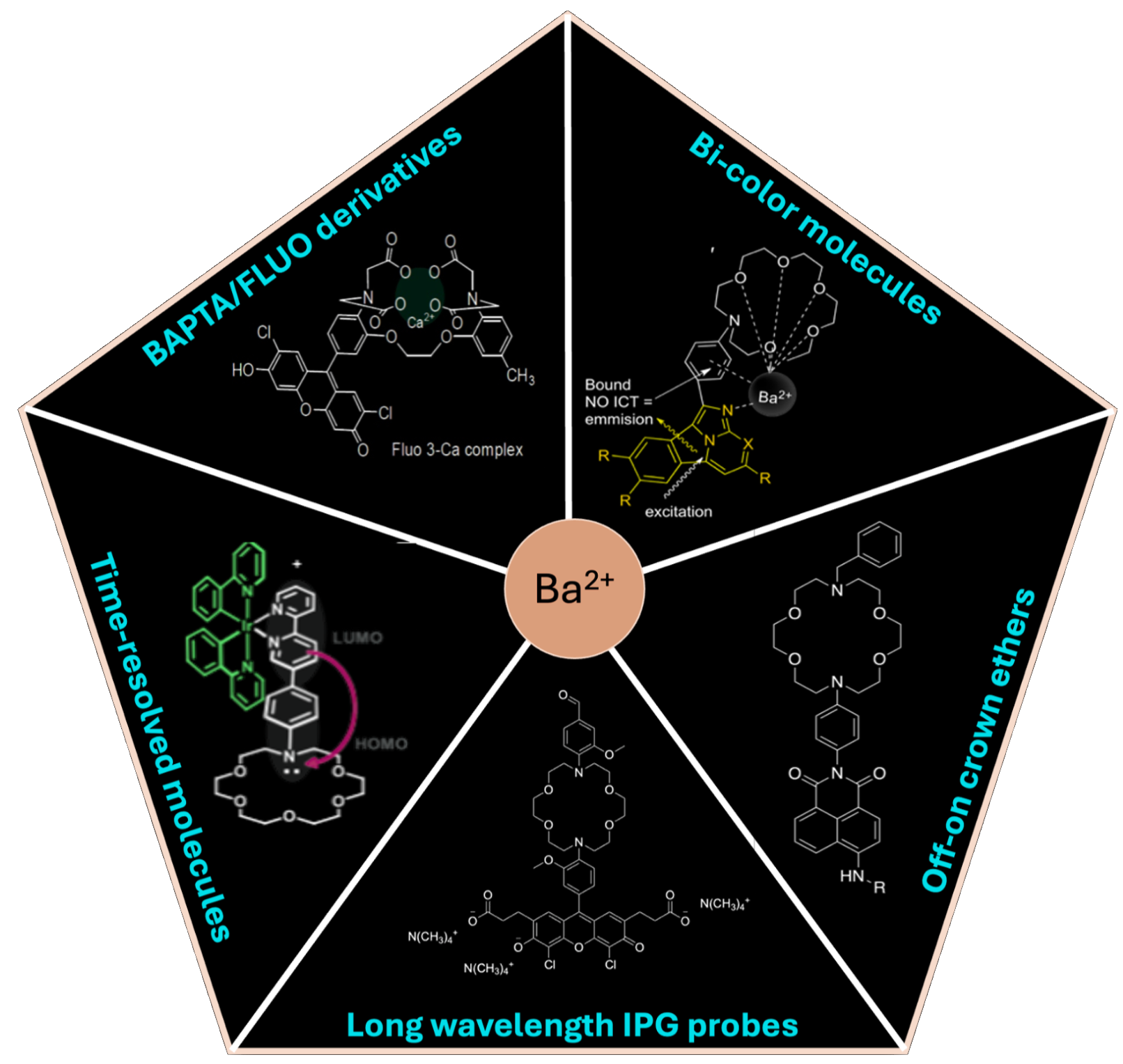
One key technological challenge for achieving barium ion identification through molecular fluorescence is the design and synthesis of appropriate molecular sensors that can work with low background and high efficiency in the working medium of a xenon time projection chamber. There is a wide space of possible molecular designs to explore, each bringing unique features and advantages.ย The figure on the left shows some of the families of molecules that have been explored by NEXT to date.ย While all of these molecules undergo changes in their fluorescence emission properties when they trap, or chelate Ba, the choice of the receptor and fluorescent group determine the selectivity and sensitivity of the molecule, and the mechanism by which it functions.
BAPTA/Fluo derivatives [1]
The first SMFI work in NEXT used molecules designed for calcium sensing for biochemical applications. These molecules use a binding domain called BAPTA coupled to a fluorescein fluorescent derivative.ย While these molecules are powerful for use in the solution-phase, in the dry conditions required by NEXT the BAPTA group cannot de-protonate to capture ions.ย The fluorescein fluorescence is also suppressed outside of solution. Thus this first generation of molecules is deemed unsuitable for the NEXT application, motivating us to develop new families of molecules for the NEXT application.
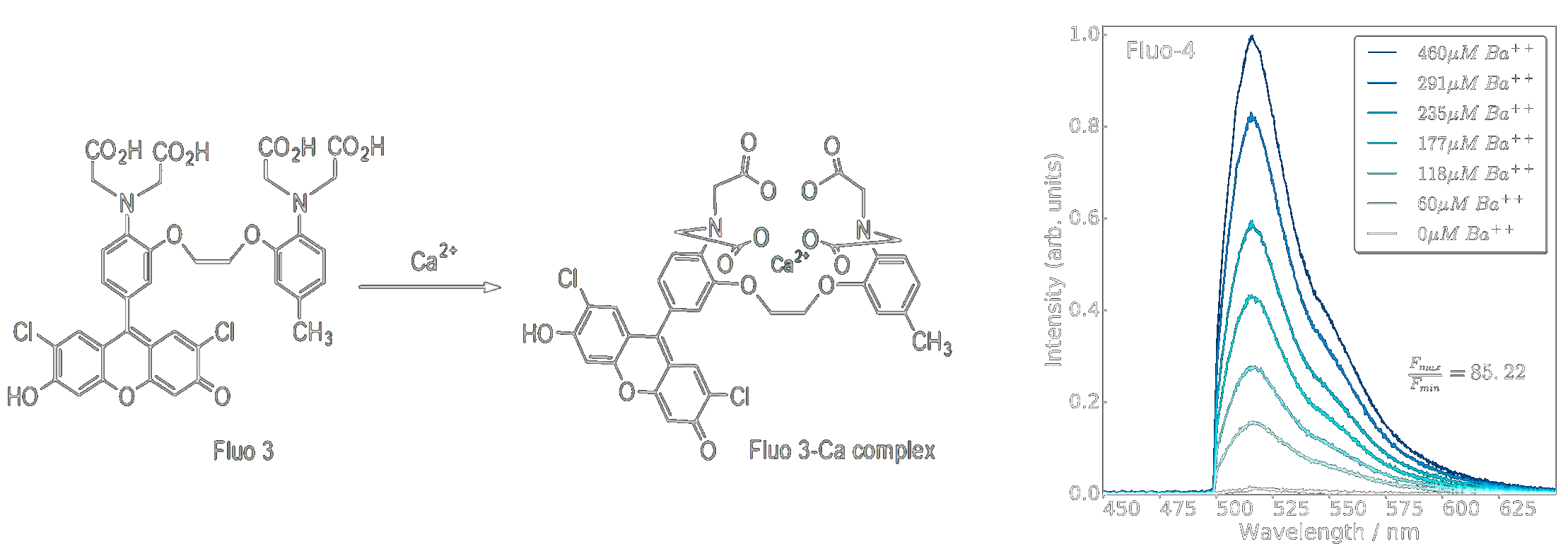
Off-on crown ethers [2, 3]
To overcome the problem of BAPTA as an unsuitable receptor, subsequent generations of NEXT barium chemosensors have been developed by NEXT collaborators using crown ethers as the binding domain. The 18c6 crown ether (a 6-unit ring made of carbon and oxygen atoms) is particularly well matched in size to Ba2+ ions.ย Coupling the crown ether to a fluorophore via a nitrogen atom leads to modulation of the fluorescence of the fluorophore.ย Variants of the crown ether itself lead to different selectivity and sensitivity to barium. Two generations of molecules have been explored, first with UV responsive fluorescent groups anthracene and pyrene [4]. and second with naphthalimide to allow the realization of single barium ion imaging in dry conditionsย [5].
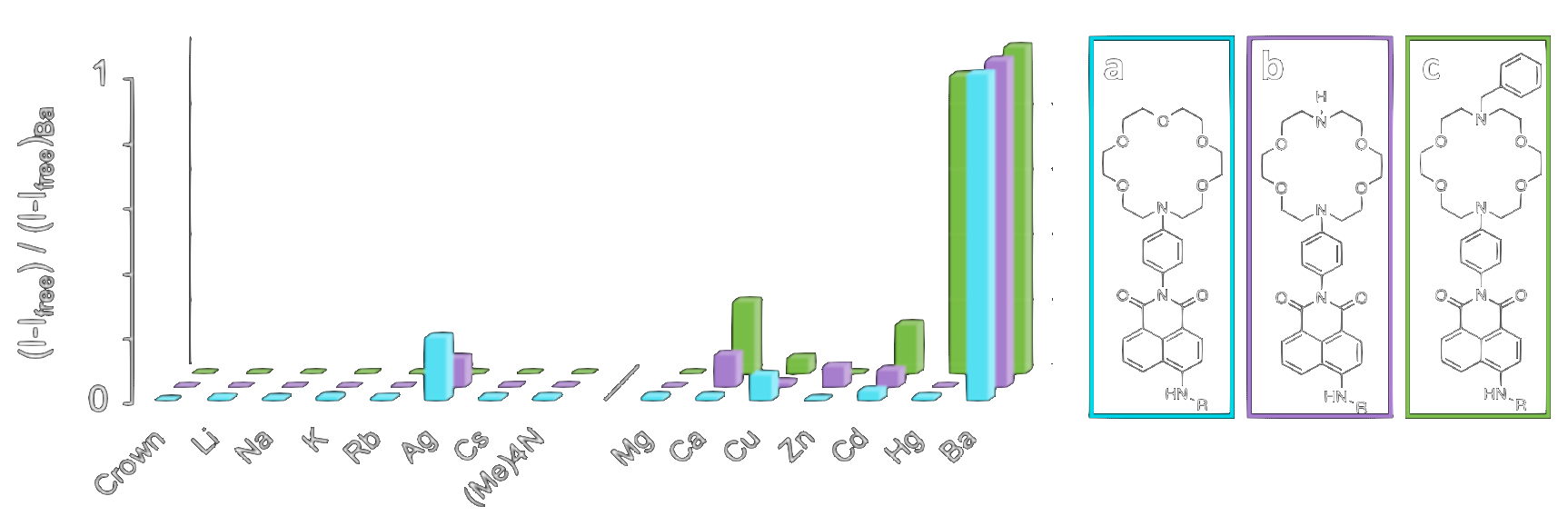
Fluorescent Bi-color Indicators (FBIs) [4]
FBIโs molecules emit green light in the free state and blue light when they trap Ba.ย Most ions only interact with the crown-ether (the head) but Ba interacts with the molecule as a whole: crown, middle ring and one N atom in the fluorophore moiety. The fluorescence emission spectra of the free molecule and the barium-bound (chelated) molecule can be seen in Fig. 3. This shift in emission means that the region around 420 nm can be used to establish the presence of Ba on chelated molecules. This offers a novel background rejection method, since a wavelength cut can be applied via an optical filter, rather than simply seeking an increase in fluorescence intensity, as exhibited by the off-on sensors. Other generations of FBI have been synthesized with the objective of increasing the color shift โฮป, and to improve the affinity for Ba2+. The second generation (FBI-G2), for example, was obtained by permuting the Ba-interacting N atom with a neighboring C atom, showing a greater color shift than the first generation. Subsequent variants exhibit a range of other properties, exploring the space of structure-function relationships within this class of sensors.
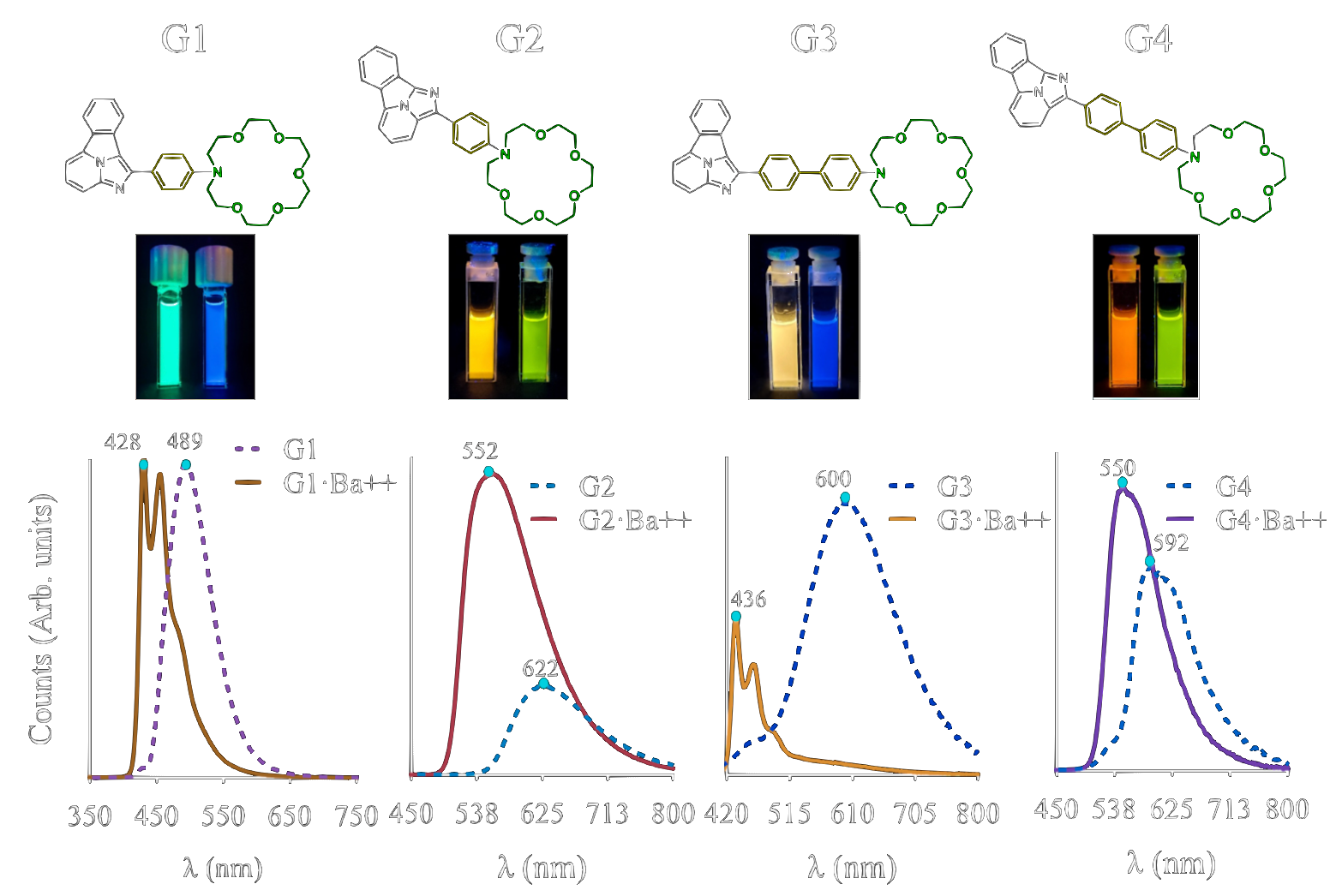
Long wavelength IPG probes [5]
Longer wavelength dyes confer significant advantages for low background single molecule fluorescence imaging, allowing experiments to reach above the autofluorescence backgrounds that are present at some level in all optical parts and substrates. The IPG family of potassium sensing probes have a binding domain strikingly similar to that developed by NEXT for barium sensing, which is no coincidence, since K+ and Ba2+ have similar ionic radii.ย The much longer wavelength fluorescence response, with emission above 500 nm, makes the IPG molecules especially amenable for single molecule fluorescence microscopy.ย The images below show dry response of IPG molecules at single ion level, when loaded with Ba2+ and imaged in dry conditions.
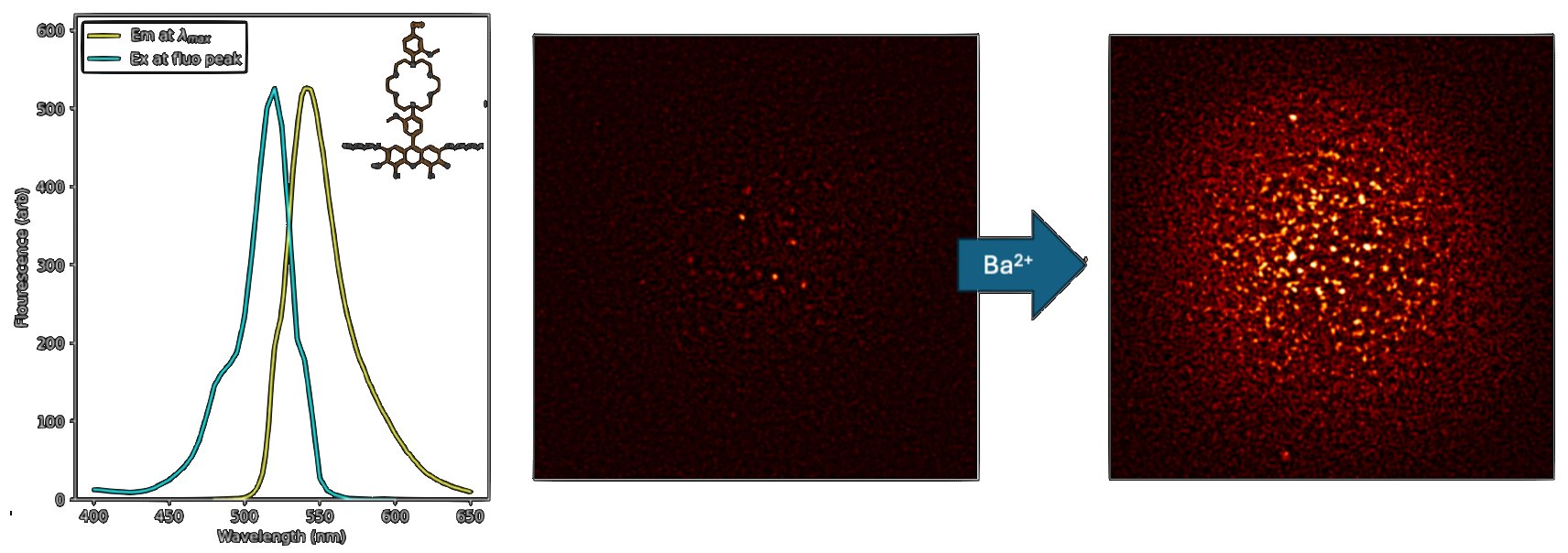
Time resolved sensors [6]
Another approach to detect the presence of Ba2+ is to exploit the different time signatures of light emission from molecules. The so-called Time-Resolved Indicators are molecules which display fluorescence emission in its free form (with a characteristic emission time of a few nanoseconds) and phosphorescence emission in its Ba-bound form (emission time around 1 millisecond or 1000 nanoseconds). This difference enables the separation between both species by a simple time cut. These TRIs are based on an inorganic complex called cyclometalated-iridium. The complex is modified to include a crown-ether moiety to act as Ba2+ receptor unit. On top of different time signatures, these molecules also display bicolor behavior in solution.ย The additional handle of time-dependent response can provide another background rejection tool, to image individual ions over autofluorescent and off-state backgrounds.
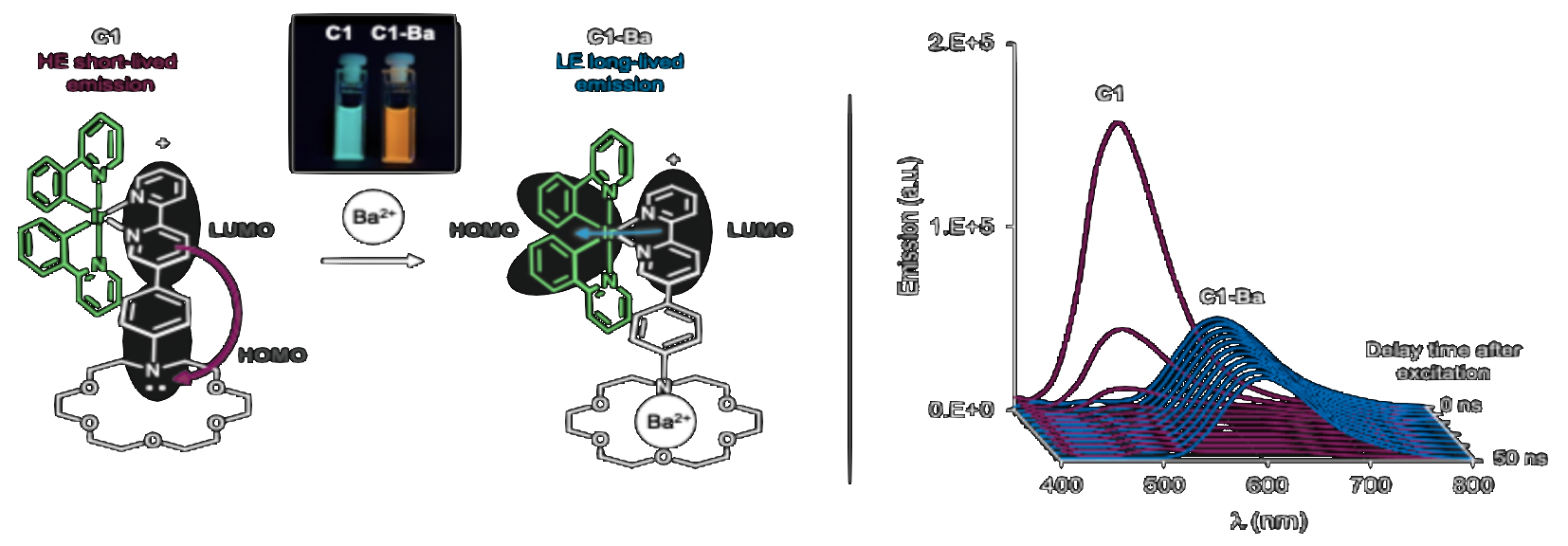
Characterizing ionic binding with surface science techniques [7]
One of the technical challenges in development of a sensor is realizing a densely packed, fully sensitive surface monolayer. A significant effort is invested in growing these molecular layers on surfaces and characterizing the response of barium sensors structurally and chemically at these surfaces. Scanning Tunnelling Microscopy (STM) provides information about the molecular conformation of barium bound and unbound surfaces, in some cases with 3D resolution across even a single molecule. The figure below shows the images obtained for a free and Ba-bound molecule evaporated on a Au (111) surface, and a model of the molecule in each condition. As can be seen, when the sensor traps Ba2+ its structure and shape change. Studying these changes in detail allows us to understanding the binding mechanism and surface interaction, and hence optimize the properties of the molecule.
STM can also measure the density of states at different positions in the molecule. In the case of FBI the HOMO-LUMO gap widens by 0.5 eV for the Ba-chelated molecule with respect to that of the free molecules. This widening is compatible with the blue shift in the absorption spectrum. Lastly, chemical effects of the chelation were characterised by X-ray Photoemission Spectroscopy (XPS). This technique yielded similar results and proved that the oxygen atoms in the crown-ether interact strongly with the Ba ion. Furthermore, the effects were found for three different molecules in the family of FBIs and for three different substrates, which supports the claim that chelation can be achieved independently of the material used to support them.
The interaction of fluorescence molecules with barium can be inferred from the structural and chemical changes that it causes. X-ray Photoemission Spectroscopy (XPS) can be used to probe the chemical changes in the atomic core levels as the dose of barium is increased. A shift of up to 0.5 eV in the oxygen 1s peak appears toward higher binding energy. This indicates that a supramolecular bond has been formed. The structure of the molecule clearly changes when it interacts with the ion, as evidenced by the images taken in Scanning Tunnelling Microscopy (STM).
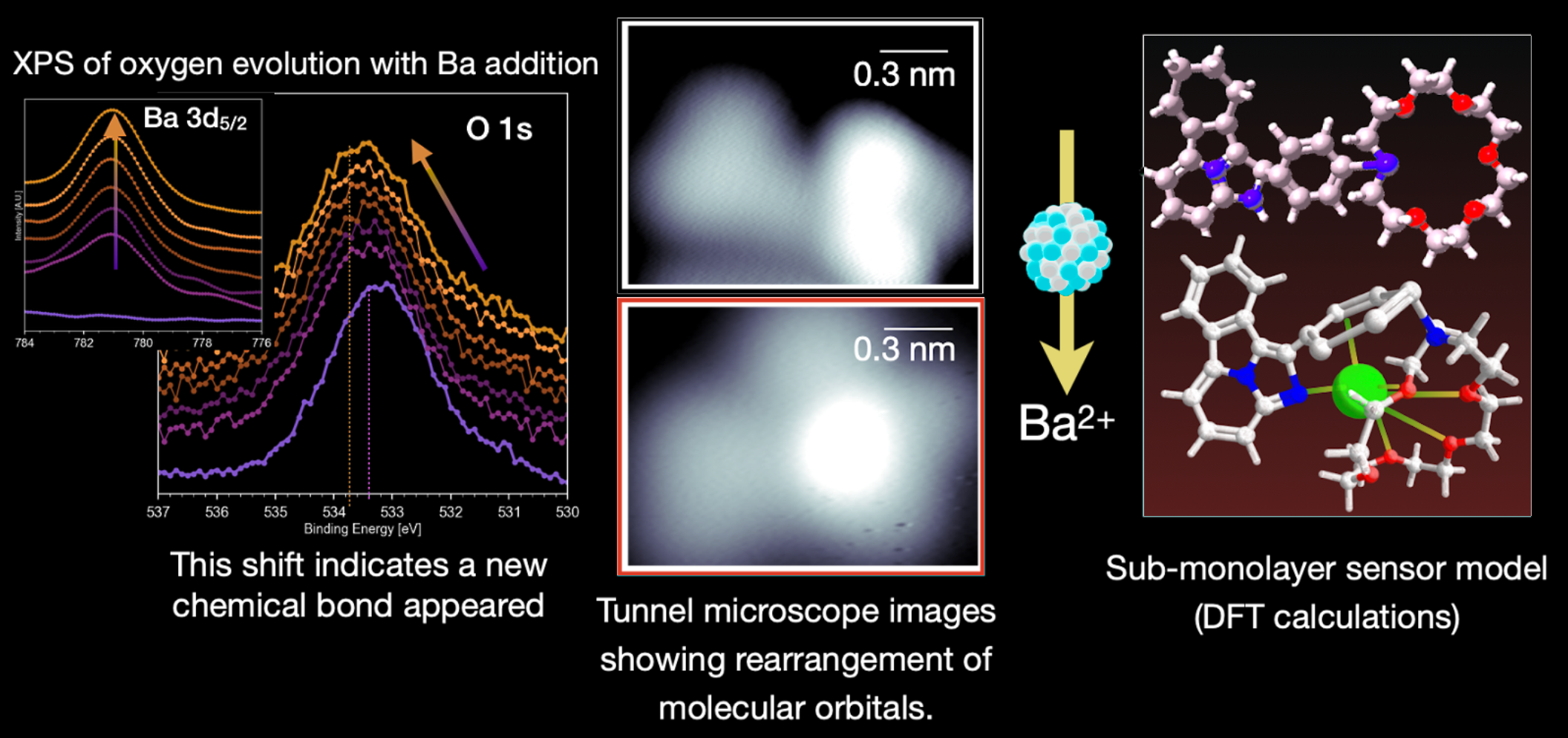
References
- A.โD. McDonald et al. (NEXT Collaboration), Phys. Rev. Lett. 120, 132504, โDemonstration of Single-Barium-Ion Sensitivity for Neutrinoless Double-Beta Decay Using Single-Molecule Fluorescence Imagingโ.
- P. Thapa et al, Sci Rep 9:ย 15097ย (2019)ย , โBarium Chemosensors with Dry-Phase Fluorescence for Neutrinoless Double Beta Decayโ
- P. Thapa et al. ACS Sensors 2021 6 (1), 192-202. โDemonstration of Selective Single-Barium Ion Detection with Dry Diazacrown Ether Naphthalimide Turn-on Chemosensorsโ.
- I. Rivilla, et al., Nature 583, 48 (2020). โFluorescent bicolour sensor for low-background neutrinoless double ฮฒ decay experiments.โ
- R. L. Miller et. al. (NEXT Collaboration), 10.26434/chemrxiv-2023-wxpbh (submitted to The Analyst), โBarium Ion Sensing with Commercial IPG K+ Molecular Probesโ
- A. I. Aranburu et al. (NEXT Collaboration)ย paper in preparation.ย
- P. Herrero, et al. (NEXT Collaboration). Nat. Comm., 13, 7741 (2022). โBa2+ ion trapping by organic submonolayer: toward an ย ultra-low background 0ฮฝฮฒฮฒ decay detector.โ