High pressure microscopy [1]
High pressure xenon gas is a complex environment in which to perform precision single molecule microscopy. Commercial microscope objectives do not function well under pressurized conditions, and the light paths in conventional microscopes do now allow for remote imaging within a vacuum or pressure vessel. The high numerical aperture optics required for single molecule sensitivity necessitate control of the focal plane position within one micron along three axes. And, the autofluorescence of various optical parts must be minimized, while managing reflections, vibrations, light noise and other confounding effects.
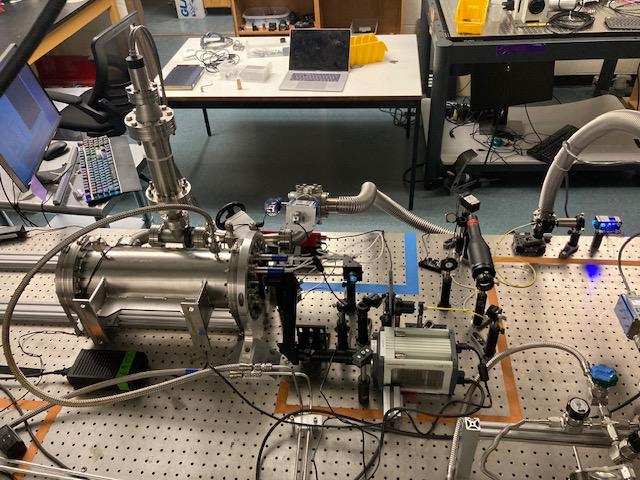
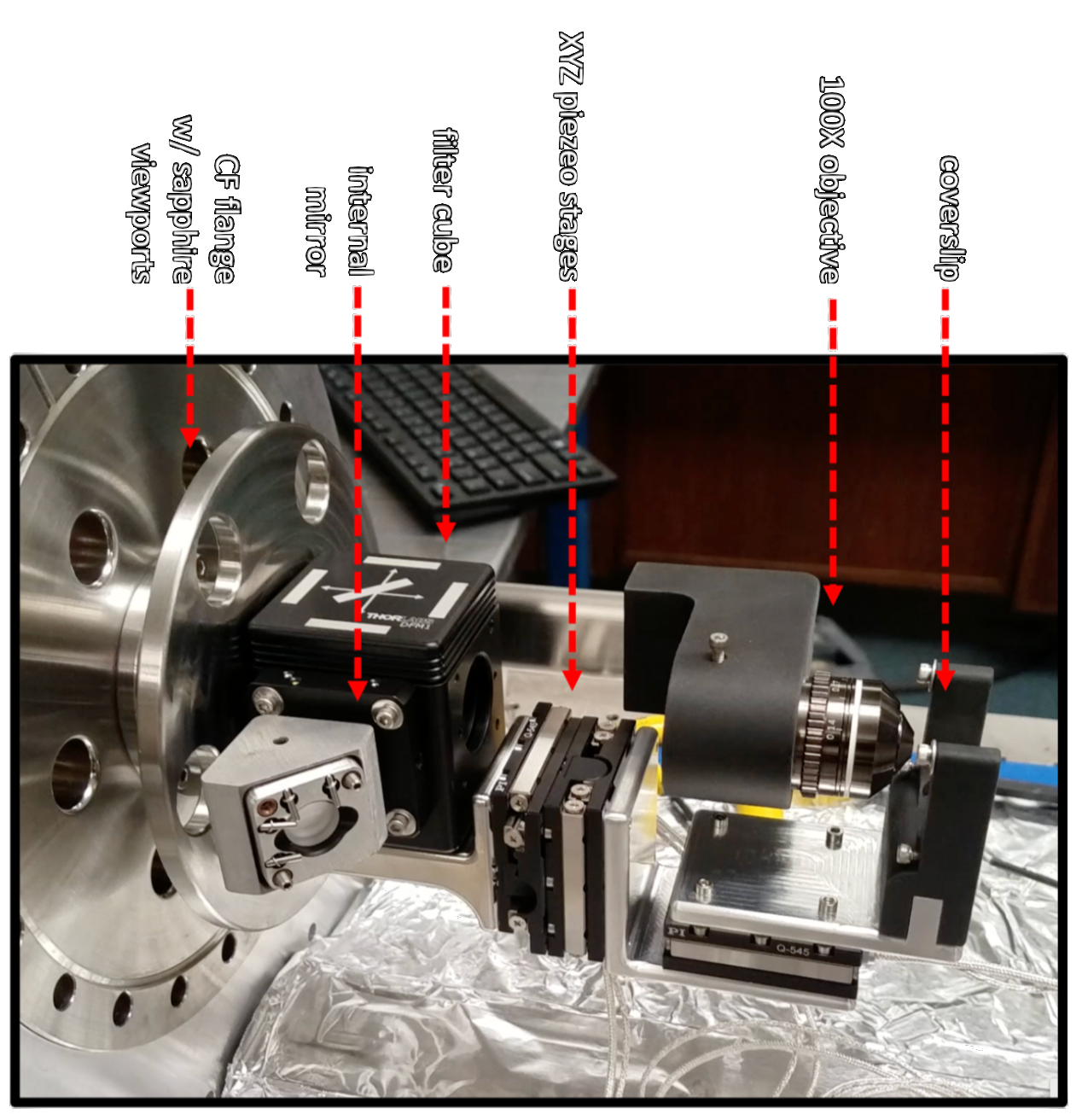
NEXT has been pursuing development of single molecule microscopy methods that can function in 10 bar of purified xenon gas. Recent advances have demonstrated imaging of individual barium ions inside a pressure chamber in these conditions, with a novel, high pressure single molecule microscope. The images below show an IPG molecular layer with and without Ba2+ ions, imaged under 10 atmospheres of xenon gas. The individual fluorescent spots are clearly resolved, and exhibit the expected single step photobleaching behavior characteristic of single molecule/ion complexes. This represents a proof of principle that single ion imaging can be accomplished in high pressure xenon gas detectors, and coupling these methods with optimized molecular monolayers and ion delivery methods will result in a full barium tagging sensor for the BOLD program.
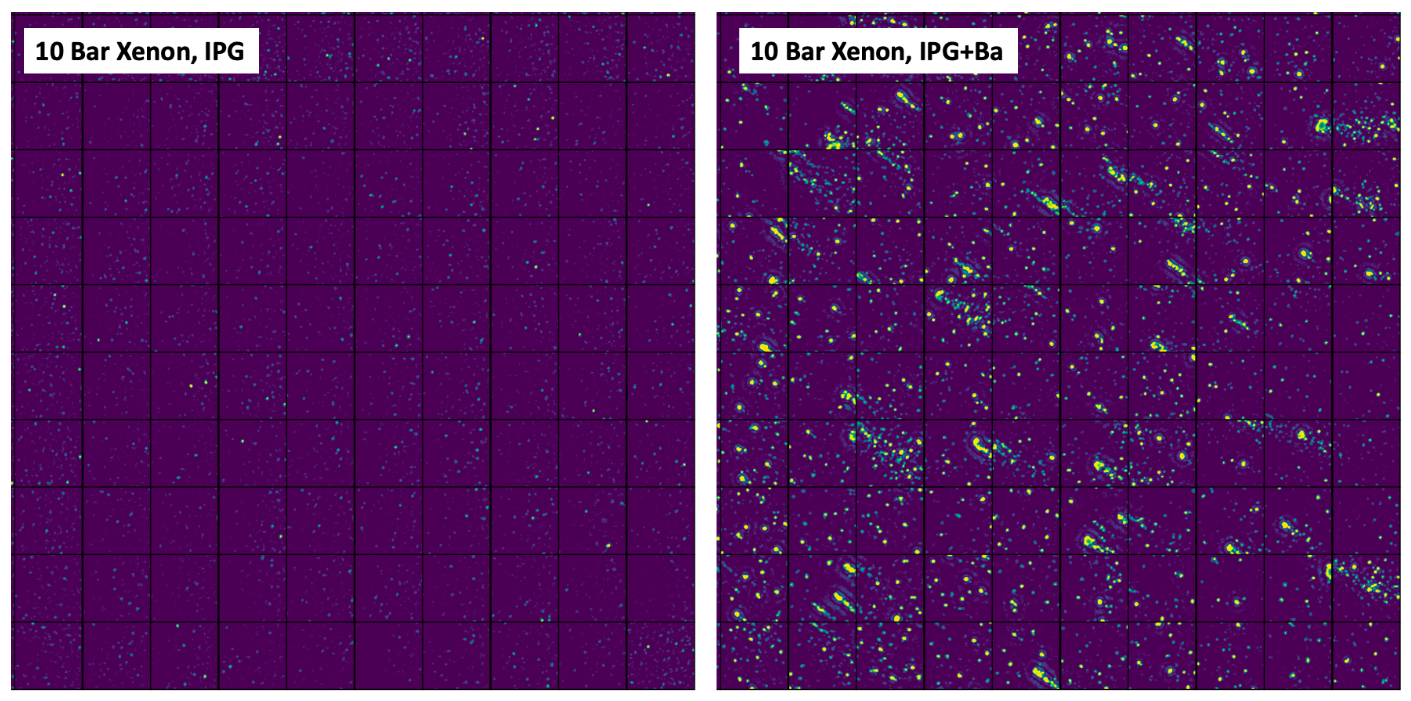
Surface monolayer growth methods [2]
One important research line in NEXT-BOLD has to do with the growth monolayer of molecular monolayers on top of substrates suitable for optical detection and implementation on the NEXT chamber. Such substrates must be transparent (to allow fluorescence detection from the back plane) and little to non-conducting (as conducting substrates quench the fluorescence). Fused silica is one of the materials that fulfills these conditions. However, many surface science characterization techniques require the samples to be conductors. Therefore, gold was chosen for the characterization stages.
Some of the considered techniques for monolayer growth intend to form a covalent bond between the fluorescent molecules and the atoms of the surface (chemisorption). These include growth of Self-Assembled Monolayers (SAM) and On-Surface Synthesis (OSS). In the present stages, the monolayers are formed by physisorption of the molecules on the surface, via Van-der-Waals bonds. Several techniques are useful in this regard: drop-casting and spin-coating are well-known techniques for surface preparation. They are carried out in air, so the production of large numbers of samples and coverage of large surfaces is feasible.ย
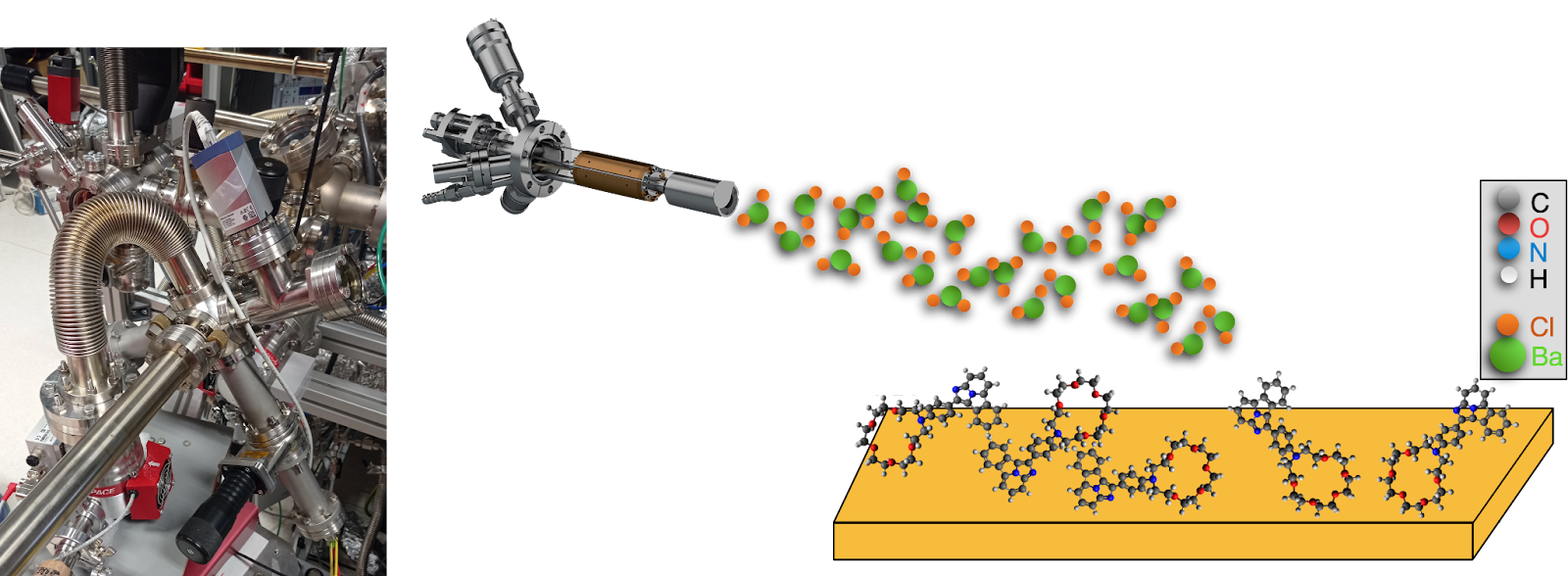
Lastly, if the molecules can be evaporated intactly, Molecular Beam Epitaxy (MBE) can also be carried out. This is the cleanest deposition method, as no solvent is involved and very high purity can be achieved in ultra-high vacuum. In this technique, the molecules are sublimated from a solid powder and the vapor reaches the substrate and sticks to it. The sublimation typically occurs at around 120-150 ยบC, for organic molecules, so the species must be able to resist those temperatures. Barium salts (BaCl2 or Ba(ClO4)2) can also be deposited on the samples in this way, so that the interaction with the molecules can be studied in-situ.ย
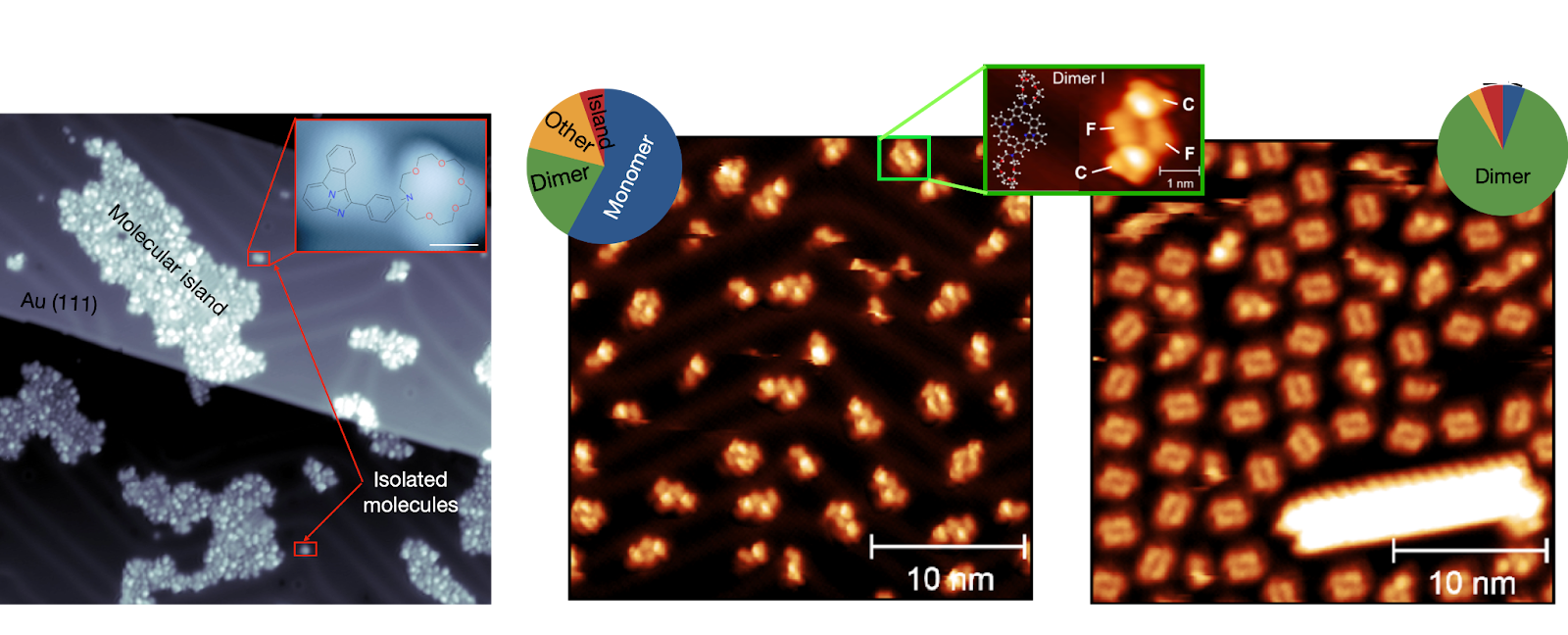
Different molecules interact differently with the substrate. The first generation of FBIs (see above) tend to form molecular islands or be isolated (monomers). The second generation (FBI-G2) tends to form mainly dimers, with a small abundance of monomers and islands. However, the relative abundance of each species changes with the absolute coverage of the surface [3].
RF carpet concentrators, and other ion collection methods [4]
The microscopy systems developed for NEXT can image few-mm2 surface areas with single molecule precision. A key challenge is then how to move the ion to this sensing volume, or move the sensor to the ion. One approach that is under intensive exploration is the use of RF carpets to guide ions to these sensors. In an RF carpet, a push field of ~20 V/cm into the carpet is counteracted by an effective potential generated by a high RF voltage applied across closely spaced electrodes. This effective RF potential causes ions to levitate over the surface, and by manipulating the applied voltages the ions can be swept toward the imaging region.
NEXT is presently pursuing R&D using current state-of-the-art PCB RF carpets with electrode pitches of 160 um, which can efficiently transport ions in xenon at pressures up to around half an atmosphere. To achieve ion transport at several bar of pressure, however, smaller electrode pitches are required. The NuBIT program within NEXT is pursuing development of ultra-fine-pitch RF carpets through microfabrication methods, with the first prototype chips at 20 nm pitch recently being delivered from the fabrication facility and moving into the testing phase.
Alternative approaches to the use of RF carpets for ion capture include mechanically actuating the sensor to the ion arrival position, or coating the full cathode in a molecular monolayer. These concepts are also under consideration for the final application in BOLD, with further study ongoing.
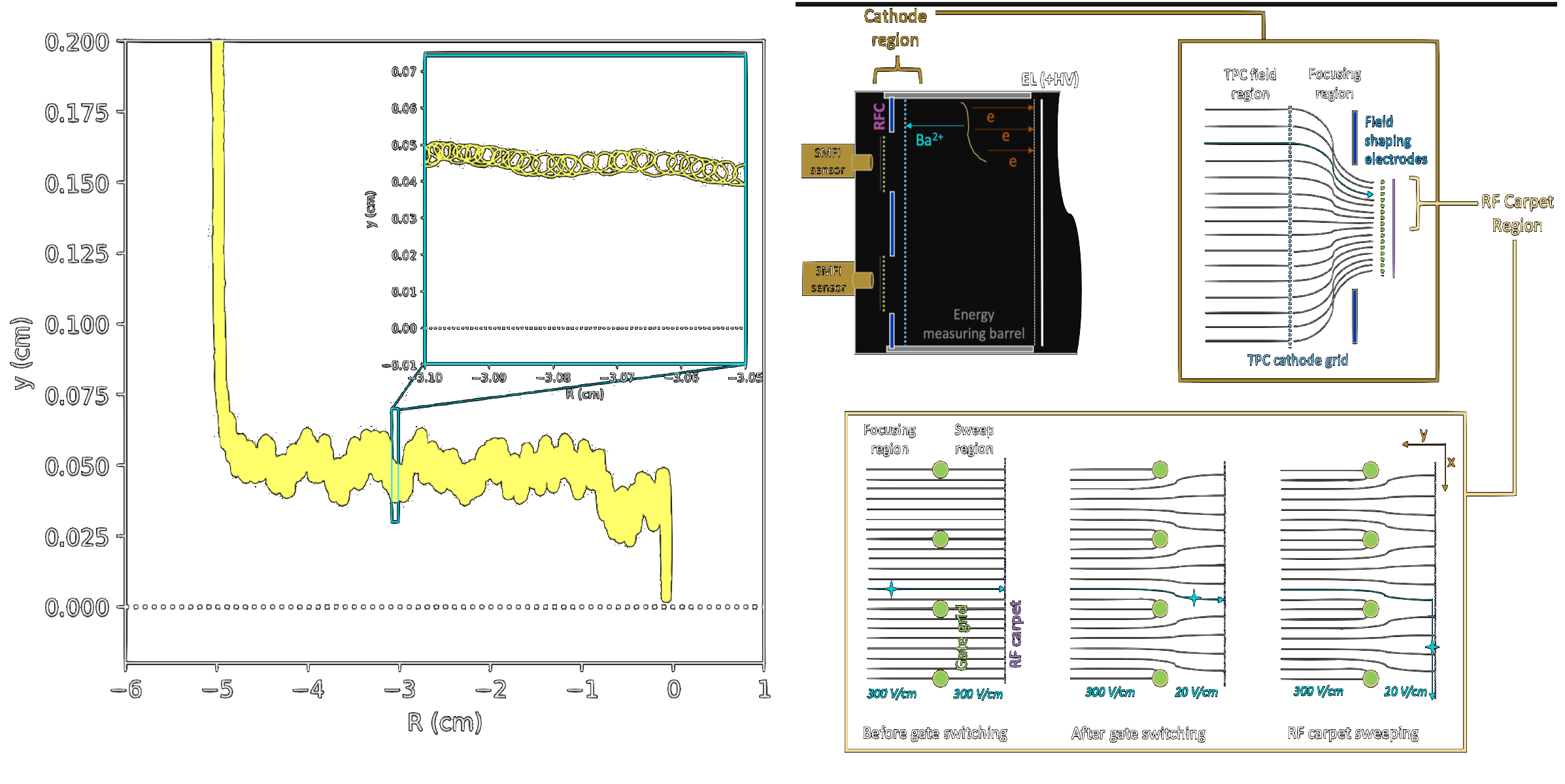
References
- N. Byrnes et al (NEXT Collaboration) (under review), โFluorescence Imaging of Individual Ions and Molecules in Pressurized Noble Gases for Barium Tagging in 136Xeโ.
- P. Herrero, et al. (NEXT Collaboration). Nat. Comm., 13, 7741 (2022). โBa2+ ion trapping by organic submonolayer: toward an ย ultra-low background 0ฮฝฮฒฮฒ decay detector.โ
- A. Yubero et al. (NEXT Collaboration) paper in preparation.
- B.J.P. Jones et al (NEXT Collaboration), Nucl Instr Meth A 1039,ย 11, 167000 (2022), โThe dynamics of ions on phased radio-frequency carpets in high pressure gases and application for barium tagging in xenon gas time projection chambersโ